Current Research Projects
"Center for Pulse Power-driven High Energy Density Plasmas"
(NNSA Stockpile Stewardship Academic Alliance Program led by Cornell University)
The Center, first established in 2002, is run under the auspices of the Cornell Laboratory of Plasma Studies in collaboration with researchers at five partner institutions: University of California, San Diego; Princeton University; Imperial College in London; Weizmann Institute of Science in Israel; and P.N. Lebedev Physical Institute in Moscow. Over the present 5 year, $11M program, the Center will investigate magnetized high-energy density plasmas for application in liner-based z-pinch fusion issues, gas-puff radiation sources, shock propagation and equation of state studies, and laboratory astrophysics through both experimental and computational studies. The UCSD group will lead the development of wire array z-pinch systems to study shockless compression and radiative shock generation in low density media, as well as carry out detailed examinations of hydrodynamic shocks in dense plasma flows where radiation loss plays a significant role.
​
​
“Characterization and Mitigation of Azimuthal Current Uniformity in Coaxial Power Feed systems relevant to MagLIF”
(Sandia National Laboratories)
​
The MagLIF scheme under study at Sandia continues to show great promise in the drive towards the demonstration of gain in inertial fusion systems. Many aspects of the design are under study through both integrated experiments and stand-alone tests. One aspect which has received little detailed attention to date is the connection of the liner to the power feed. Several mounting methods have been used, including a ‘slip-fit’ design which has the potential to leave a small (25mm) vacuum gap all the way around the liner as it is recessed into the electrode. The design is intended to mitigate axial compression of the liner as the power feed is put under vacuum, but is important to determine if potential issues could arise from the presence of such a gap in MagLIF and future load designs. It is also important to note that there are many conductor-conductor and conductor-insulator interfaces in a large pulsed power systems, and any effects are likely to be strongly relevant to these areas too.
Our work over the last few years has investigated the likely effect a vacuum gap in the power feed has on the current distribution in a coaxial power feed or liner. Data from both low current (100s A) and high current (1MA) experiments shows extended time period over which current non-uniformity is maintained, and which will impact full-scale implosion systems. Methods of mitigation including electrode coatings and the application of Bz field both dhow strong promise, and the project continues to investigate these on present and up-coming pulsed power drivers.
​
​
​
​
“A Portable Thomson Scattering System for HED Plasmas.”
(ARPA-E, Lawrence Livermore National Laboratory)
​
This project is developing an optical (532nm) Thomson Scattering system for deployment on a range of fusion experiments funded by ARPA-E. Presently, the system is in operation at the FuZe generator at Zap Energy in Everett, WA. The OTS diagnostic measures both electron temperature and electron density in a localized volume (~.05mm^3) at 17 positions in the plasma simultaneously with 1.5ns temporal resolution
​
​
​
​
​
​
​
​
​
“Investigation of laser-produced plasmas as a soft x-ray source for metrology in silicon wafer systems.”
(KLA-Tencor Corporation)
​
Investigation into EUV sources using nobel gas targets to develop novel laser-produced plasma sources for industrial implementation.
​
​
​
​
​
​
​
​
​
​
​
Recent Projects
​
"An Investigation of the Effects of magnetic Fields and Collisionality on Shock Formation in Radiatively Cooled Plasma Flows"
(Dept of Energy, Office of Fusion Energy Sciences)
The development and behaviour of shocks is fundamental to the evolution of many systems, particularly in astrophysics, and includes phenomena such as the Earth's magnetosphere, supernovae and the generation of cosmic rays. Radiative cooling, magnetic fields and collisionality all play a role, and the interplay of these drives the observable phenomenon. High radiation loss allows an increase in the density jump across a shock relative to the adiabatic case, and may lead to new instabilities observed only in highly compressible flows. Embedded magnetic fields limit fluid compressibility, but the combination with high radiation loss dynamically changes the magnetic Reynolds number at the shock front. In systems with long particle mean free paths, these process dominate over coulomb collisions and localization of the mass density occurs through interaction with the magnetic field structure. The focus of this proposal is to carefully evolve a test-bed for the study of shock formation and evolution relevant to many of the above issues. This will be based on pulsed power-driven ablating wire experiments supported by parallel computational studies. The program will progress from investigating hydrodynamic (collisional) only shock systems, to collisional shocks with a significant B-field, and finally to collisionless, magnetized shocks.
​
​
​
"Resolving the Issue: The Dynamics of Magnetized Astrophysical Jets through Pulsed Power HEDP Laboratory Studies"
(DoE/NNSA High Energy Density Laboratory Plasmas Program)
This collaboration, known as JetPAC (Jets: A Plasma and Astrophysics Collaboration), is led by Prof. Adam Frank at Rochester University, and involves the UCSD Pulsed Power Plasmas and HEDP Groups, the Plasma Physics Group at Imperial College London, and Rice University, TX. The aim of the project is to more directly design and carry out experimental studies of plasma jets which have relevance to astrophyical jet formation and propagation, particularly those emanating from Young Stellar Object (YSOs). The project is unique in several ways. For the first time, pulsed power experiments will be carried out (Imperial, UCSD) with computational support from both the plasma (Imperial) and astrophysics (Rochester) communities, and will be guided by observational needs (Rice). The project has also secured shot time at the 26 MA Z machine at Sandia National Laboratories which will generate jets of unprecedented density and Reynolds number, and push experiments significantly further into the turbulent and astrophyically relevent regimes.
​
​
"Investigation of current diffusion and heat transport in magnetically driven implosions"
(DoE/NNSA High Energy Density Laboratory Plasmas Program)
The project will examine two areas of basic physics in magnetized high energy density (HED) imploding pinch plasmas, in collaboration with Alameda Applied Science Corp. (AASC): Firstly, the effect of instability growth at an implosion surface on magnetic field diffusion, and secondly the effect of an applied axial B-field on radial heat loss through examination of the pinch temperature.
The configuration to be utilized is that of the dense plasma focus (DPF). This provides an excellent opportunity to examine HED issues in a system with well-defined initial conditions, and where an implosion surface impinges on a static gas fill. In addition, an inherently high repetition rate (~0.1 Hz) will give meaningful statistics on the current distribution in loads where the radiative cooling rate is readily varied. Whilst the total current rise-time is long (~1μs) the radial compression phase occurs in ~100ns with velocities ~20 cm/μs and so is directly relevant to many IFE implosion systems. Whilst the densities in the pinch are low, this provides an opportunity to more easily access the low plasma beta regime, since B-fields of order < 1 T are sufficient to limit the radial electron transport. The experimental program will be supported by 3-dimensional Magneto-Hydrodynamics (MHD) simulations which will provide detailed comparisons to the experimental work.
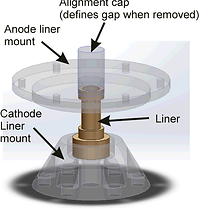
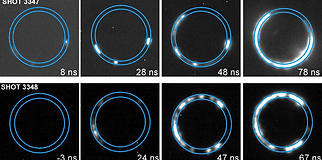
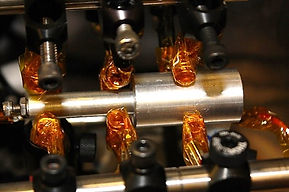



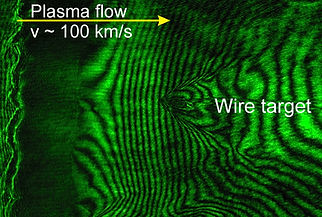
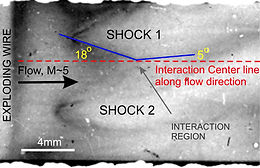
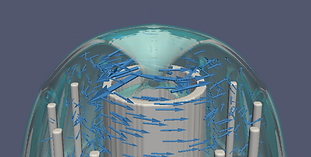